Water is the lifeblood of any agricultural system, and its quality plays a crucial role in determining the success of plant production. For greenhouse and indoor agricultural operations, the challenge of maintaining water quality has spurred interest in advanced technologies. One such innovation is nanobubble technology, which is now gaining traction in water treatment.
Nanobubbles, tiny gas bubbles less than 200 nanometers in diameter, have been found to possess unique properties that make them highly effective for various applications, including commercial water treatment. Unlike regular bubbles, nanobubbles exhibit long-term stability, meaning they can remain suspended in water for extended periods.
This property, along with their high surface-area-to-volume ratio, allows for enhanced mass transfer of gases like oxygen or ozone into the water. Nanobubbles can be produced through various methods, but in commercial applications, their production in bulk is usually achieved via either electrolysis, membranes, or cavitation(Favvas et al., 2021).
In agricultural water systems, nanobubbles can provide numerous benefits:
- Improved Oxygenation/Ozonation: The high surface area of nanobubbles facilitates efficient gas transfer, allowing a greater quantity of gas to be dissolved in solution. This improves disinfection and aeration, promoting healthy plant root development and improving nutrient uptake.
- Biofilm Reduction: Nanobubbles have been shown to help break down biofilms, which are thin layers of bacteria that accumulate on surfaces in water systems. Biofilms can harbor harmful pathogens and impede water flow, leading to disease and clogged emitters.
- Water Clarification: Nanobubbles can act as micro-flocculants, helping to remove suspended solids and impurities in water. This ensures cleaner water for irrigation, reducing the risk of clogged systems and enhancing plant growth.
Ozone Nanobubbles: An Enhanced Water Treatment Solution
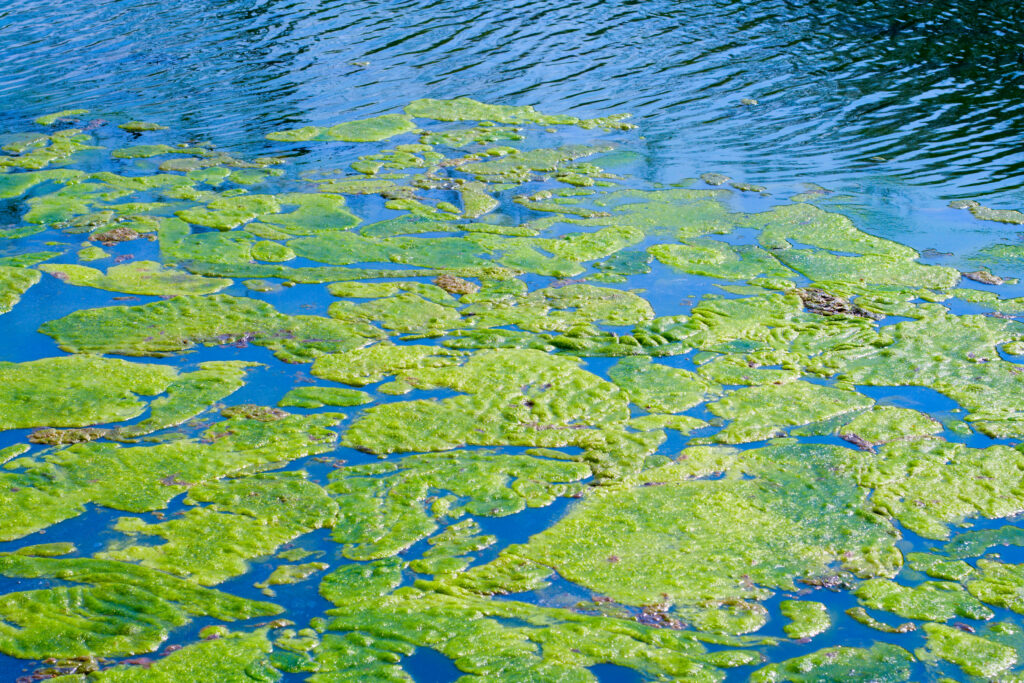
While nanobubbles themselves offer significant benefits in water treatment, they are most commonly employed with oxygen which is a weak oxidant. The combination of ozone with nanobubble technology opens a new frontier by providing vastly improved water treatment, particularly in greenhouse and indoor agricultural production.
Ozone, a powerful oxidizing agent, has long been used for water treatment due to its ability to destroy a wide range of microorganisms, break down organic matter, and eliminate harmful contaminants. When introduced in the form of nanobubbles, ozone’s efficacy is significantly enhanced.
The smaller size and stability of ozone nanobubbles, in comparison to traditional methods, allow for more effective diffusion and interaction with contaminants in water. In greenhouses and indoor agriculture, this can lead to:
- Pathogen Control: Ozone nanobubbles are highly effective at inactivating pathogens, including those commonly found in greenhouse or indoor agriculture such as Pythium, Phytophthora, and Fusarium. The enhanced reactivity and residual times of ozone in nanobubble form ensure that these pathogens are eliminated more rapidly and thoroughly than with traditional ozone treatment.
- Reduced Chemical Usage: By using ozone nanobubbles, growers can reduce their reliance on chemical disinfectants, which may leave harmful residues and negatively affect plant health. Ozone breaks down into oxygen, leaving no harmful byproducts.
- Sustainable Water Reuse: In closed-loop systems common in greenhouse production, maintaining high water quality is essential for reusing irrigation water. Ozone nanobubbles can eliminate pathogens even under high-flow situations, oxidize organic contaminants, and reduce biofouling, allowing for the safe recirculation of irrigation water.
Scientific Backing – Greenhouse Production
Recent studies have delved into the specific benefits of ozone and nanobubble technology in agriculture. For instance, a study by (Zhao et al., 2024) explored the use of ozone nanobubble in hydroponically cultivated lettuce, demonstrating improved plant growth and reduced microbial contamination. Studies have investigated disinfection efficacy using ozone micro- and nano-bubbles in industries such as agriculture, wastewater, and drinking water, finding that small bubble sizes significantly improve the oxidative capacity of ozone and residual times, leading to better microbial inactivation and contaminant removal compared to conventional ozonation methods (Seridou & Kalogerakis, 2021).
There is debate in the scientific literature regarding phytotoxicity resulting from ozonation of irrigation water for greenhouse crops, however, evidence suggests that direct application to plants can safely achieve pathogen control provided a) intermittent dosage strategies are used to minimize oxidative stress to the plants and b) that loss of iron and manganese as a result of ozonation is accounted for in the fertigation strategy (Graham et al., 2011, 2012; Ishii et al., 2022; Martínez-Sánchez & Aguayo, 2019; Tahamolkonan et al., 2022; Tamaki et al., 2020; Zheng et al., 2020).
There is thus strong potential for combining the use of ozone and nanobubble technology in the treatment of irrigation water, providing powerful oxidation with improved residual times and offering a safe alternative to conventional pesticides. Because ozone decomposes into oxygen, crop health is further enhanced through high dissolved oxygen concentrations and the introduction of chemical residues can be avoided.
Scientific Backing – Other Industries
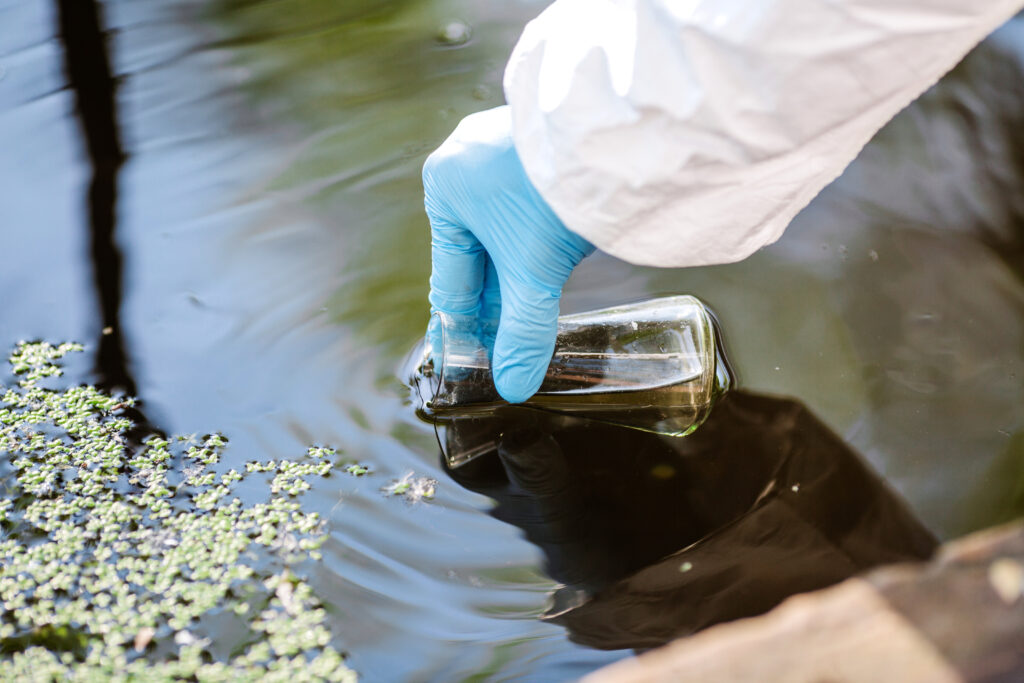
Ozone and nanobubbles have both been studied extensively for use in water treatment, in the context of various industries such as wastewater, potable water, aquaculture, ground remediation, and other applications (Davidson et al., 2021; Gonçalves & Gagnon, 2011; Khan et al., 2020; Lei et al., 2023; Powell & Scolding, 2018; Spiliotopoulou et al., 2018).
Ozone is a powerful oxidant with excellent disinfection properties, and is oftentimes combined with catalysts that degrade it rapidly to produce hydroxyl radicals at high concentrations; hydroxyls are particularly useful for the removal of complex organic molecules or other contaminants that are resistant to other treatment methods. In the context of ponds, lagoons, and lakes, the short half-life of ozone is a limiting factor as diffusion of the gas throughout entirety of water bodies can be limited by the residual time of the oxidant.
By employing the use of nanobubbles <200nM in size, a unique phenomenon occurs where the gas bubbles become negatively or non-buoyant, instead drifting randomly throughout the solution via Brownian Motion. This unique property is particularly useful for diffusion across large, deep bodies of water. These dispersal mechanisms are attributed to a higher internal pressure of the gasses inside the bubbles and a more negatively charged surface, overcoming the forces traditionally associated with bubbles in water(Khan et al., 2020).
While the underlying mechanisms are still debated, there is growing consensus that nanobubbles provide a significant advantage in various water treatment applications, especially with gasses such as oxygen or ozone where solubility and dispersal are limiting factors.
Conclusion
Nanobubbles, and specifically ozone nanobubbles, represent a transformative approach to water treatment in agricultural water treatment, and other industries. By leveraging the enhanced properties of these tiny bubbles, growers and operators can ensure superior water quality, reduce chemical inputs, improve water use efficiency, and improve productivity. The peer-reviewed research supporting this technology underscores its potential to revolutionize commercial water management in sustainable agriculture and general water treatment, making it a compelling solution for a future where water is becoming increasingly scarce.
References:
Davidson, J., Summerfelt, S., Espmark, Å. M. O., Mota, V. C., Marancik, D., Earley, R. L., Snead, A., & Good, C. (2021). Effects of ozone on post-smolt Atlantic salmon (Salmo salar) performance, health, and maturation in freshwater recirculation aquaculture systems. Aquaculture, 533. https://doi.org/10.1016/j.aquaculture.2020.736208
Favvas, E. P., Kyzas, G. Z., Efthimiadou, E. K., & Mitropoulos, A. C. (2021). Bulk nanobubbles, generation methods and potential applications. In Current Opinion in Colloid and Interface Science (Vol. 54). Elsevier Ltd. https://doi.org/10.1016/j.cocis.2021.101455
Gonçalves, A. A., & Gagnon, G. A. (2011). Ozone application in recirculating aquaculture system: An overview. Ozone: Science and Engineering, 33(5), 345–367. https://doi.org/10.1080/01919512.2011.604595
Graham, T., Zhang, P., & Dixon, M. A. (2012). Closing in on upper limits for root zone aqueous ozone application in mineral wool hydroponic tomato culture. Scientia Horticulturae, 143, 151–156. https://doi.org/10.1016/j.scienta.2012.06.003
Graham, T., Zhang, P., Woyzbun, E., & Dixon, M. (2011). Response of hydroponic tomato to daily applications of aqueous ozone via drip irrigation. Scientia Horticulturae, 129(3), 464–471. https://doi.org/10.1016/j.scienta.2011.04.019
Ishii, M., Lam, V. P., Fujiwara, K., & Park, J. S. (2022). Intermittent Root Flushing with Ozonated Water Promotes Growth of Japanese Mustard Spinach ( Brassica rapa var. perviridis) grown in a Nutrient Film Technique Hydroponic Culture—Preliminary Results. Ozone: Science & Engineering, 44(5), 464–472. https://doi.org/10.1080/01919512.2021.1967723
Khan, P., Zhu, W., Huang, F., Gao, W., & Khan, N. A. (2020). Micro-nanobubble technology and water-related application. Water Science and Technology: Water Supply, 20(6), 2021–2035. https://doi.org/10.2166/ws.2020.121
Lei, H., Wang, W., Liang, Y., Xiao, Z., Pan, H., Wang, L., & Du, M. (2023). Effect of Nano-Bubble Irrigation on the Yield and Greenhouse Gas Warming Potential of Greenhouse Tomatoes. Agronomy, 13(12). https://doi.org/10.3390/agronomy13122917
Martínez-Sánchez, A., & Aguayo, E. (2019). Effect of irrigation with ozonated water on the quality of capsicum seedlings grown in the nursery. Agricultural Water Management, 221, 547–555. https://doi.org/10.1016/j.agwat.2019.05.027
Powell, A., & Scolding, J. W. S. (2018). Direct application of ozone in aquaculture systems. In Reviews in Aquaculture (Vol. 10, Issue 2, pp. 424–438). Wiley-Blackwell. https://doi.org/10.1111/raq.12169
Seridou, P., & Kalogerakis, N. (2021). Disinfection applications of ozone micro- And nanobubbles. In Environmental Science: Nano (Vol. 8, Issue 12, pp. 3493–3510). Royal Society of Chemistry. https://doi.org/10.1039/d1en00700a
Spiliotopoulou, A., Rojas-Tirado, P., Chhetri, R. K., Kaarsholm, K. M. S., Martin, R., Pedersen, P. B., Pedersen, L. F., & Andersen, H. R. (2018). Ozonation control and effects of ozone on water quality in recirculating aquaculture systems. Water Research, 133, 289–298. https://doi.org/10.1016/j.watres.2018.01.032
Tahamolkonan, M., Ghahsareh, A. M., Ashtari, M. K., & Honarjoo, N. (2022). Tomato (Solanum lycopersicum) growth and fruit quality affected by organic fertilization and ozonated water. Protoplasma, 259(2), 291–299. https://doi.org/10.1007/s00709-021-01657-7
Tamaki, M., Ikeura, H., & Enmei, N. (2020). Growth response of hydroponic leaf lettuce and komatsuna to ozone microbubble treatment. Journal of Plant Nutrition, 43(10), 1369–1377. https://doi.org/10.1080/01904167.2020.1730896
Zhao, Q., Dong, J., Li, S., Lei, W., & Liu, A. (2024). Effects of micro/nano-ozone bubble nutrient solutions on growth promotion and rhizosphere microbial community diversity in soilless cultivated lettuces. Frontiers in Plant Science, 15. https://doi.org/10.3389/fpls.2024.1393905Zheng, L., Liu, C., & Song, W. (2020). Effect of Ozonated Nutrient Solution on the Growth and Root Antioxidant Capacity of Substrate and Hydroponically Cultivated Lettuce (lactuca Sativa). Ozone: Science and Engineering, 42(3), 286–292. https://doi.org/10.1080/01919512.2019.1668256